Contributed by Aqeel Ahmed
The writer is a postdoctoral researcher in theoretical particle physics. He can be reached at his Twitter handle @aqeelhmed.
There are four fundamental forces in nature, namely, electromagnetism, weak nuclear force, strong nuclear force and gravity. Electromagnetism can be observed in daily life experience for instant lightning is a natural phenomenon. Weak nuclear force is manifested in radioactivity which is used to cure cancer. Strong nuclear force, on the other hand, is responsible for keeping the nucleus (protons and neutron) together. Whereas, the gravity is observed in everyday life, for example, the planets of solar system revolve around the sun due to gravity.
It is important to note that electromagnetism and gravity are long range forces, i.e., these forces can act for longer distances, whereas, weak and strong nuclear forces are only manifested at very short distances, i.e., of the size of a nuclei which is about 10-15 meters. Gravity is very weak as compared to other three fundamental forces and it only manifests itself on massive objects. Since the masses of fundamental particles like electrons, quarks (which are the building blocks of protons and neutrons) are very small therefore they don’t feel gravity as compared to the strength of others three forces. Hence in the study of the properties of elementary particles physicist normally don’t consider the gravity, therefore, we are left with three forces, electromagnetism, weak and strong nuclear forces.
All the forces described above are mediated between the particles by force carrier particles called “gauge boson”. For example the electromagnetic forced between the two electrons is mediated by “photons”. One can simply think this phenomenon in a naive example of badminton, as the force between the two badminton players is mediated by a “shuttlecock” similarly all the fundamental forces are mediated by the gauge bosons. Since there are four fundamental forces in nature therefore the “gauge bosons” are of four different kinds, depending on the nature of force their properties are different. For our purpose to understand the role of Higgs field/boson only two forces are important, namely the electromagnetism and the weak nuclear force.
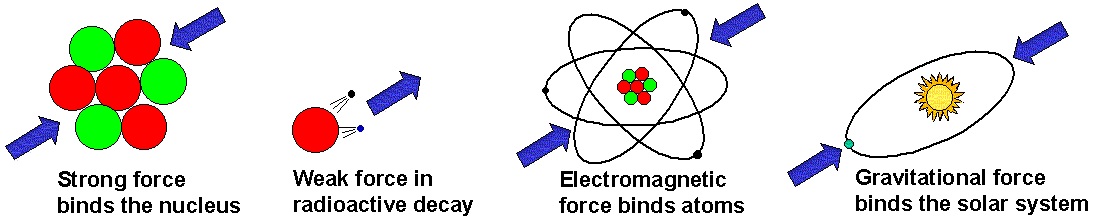
As we pointed out that electromagnetism is a long range force, i.e., it can be felt between the charged particles (say two electrons) even if they are separated by large distances, therefore the “shuttlecock” (the photons) should be massless. Hence we can say that electromagnetism is long range force because its force carriers (photons) are massless. Now we consider the weak nuclear force, as we said that this force is very short range force, i.e., it only acts below the size of a nuclei which is about 10-15 meters. By the analogy of shuttlecock you can answer the puzzle that why the weak force is short range? The answer is simple that if we make the shuttlecock (the force carrier particles) very heavy then the shuttlecock will only travel a little distance. Therefore we can say that force carries “gauge bosons” of weak nuclear force are massive, hence it is a short range force. These gauge boson are called W+ and W-, where +,- is the electric charge on these bosons.
In particle physics one of the most important guiding principle are “symmetries” (global/gauge). The (global) symmetries are in general complicated but to explain in a simple way one can think of rotational symmetry. For example if we take a sphere (say a football) and rotate it in any direction it looks the same as it was before rotation. Therefore we can say that a sphere is “symmetric” under rotation. In particle physics language we rephrase this fact that this object (sphere) is invariant (symmetric) under certain transformations (rotation). If a theory is invariant (symmetric) under certain transformations then it means that the theory will give same results if it is transformed (say rotated).
If we have a gauge symmetry in a theory, we require that the theory should be gauge invariant (gauge symmetric) under certain gauge transformations. It turns out that all the theories which have massless force carriers (gauge bosons) are gauge invariant under certain gauge transformations for example electromagnetism. Whereas, the theories which have massive force carriers (gauge bosons) are not gauge invariant for example weak interactions. It was one of the biggest puzzle in 1950s and early 1960’s for theoretical particle physicists that how to make weak theory a nice gauge invariant theory which has massive gauge bosons?
Since the theories with massive gauge bosons are not gauge invariant which means that the symmetries are broken. Particle physicists at that time in particular Nambu [1] and Goldston [2] independently have studied the effects of broken symmetries in the global symmetric theories (a global symmetry can be promoted to make a gauge symmetry by introducing gauge fields). Goldston proposed a theorem [2] that if a global symmetry is “spontaneously” broken then there should be massless scalars (which has no preferred direction in space spin 0 particle). One year later Goldstone, Salam and Weinberg [3] proved the Goldston theorem that the broken symmetries give massless scalar particles, called Goldston bosons, which was a great result at that time. But still it does not solve the problem of how to give masses to gauge bosons.
In 1964 Englert and Brout wrote in their seminal paper [4] and few weeks later Higgs [5] also got the same results independently. In their papers they showed that if the gauge symmetry is broken spontaneously then the massless gauge bosons acquire mass by eating the massless Goldstone boson. This solves the outstanding problem of how to give masses to gauge bosons. For the idea of spontaneous symmetry breaking now called Brout-Englert-Higgs (BEH) mechanism or simply Higgs mechanism. Englert and Higgs were awarded Nobel prize in physics in 2012 (Unfortunately Brout died in 2011 and could not share the prize). It is important to mention that few weeks later the papers by Englert-Brout and Higgs, Professor Salam’s group at Imperial College (Guralnik, Hagen and Kibble [6]) also obtained the similar results independently and their paper is more comprehensive in its implications. In recognition of their outstanding work J. J. Sakurai Prize for Theoretical Particle Physics by American Physical Society was awarded to Brout, Englert, Higgs, Guralnik, Hagen and Kibble in 2010.
After the BEH mechanism of giving masses to the gauge bosons (force carriers) was established, in 1967 Steven Weinberg [7] and Abdus Salam [8] independently proposed a model which unifies the electromagnetic force with the weak nuclear force. Salam termed this theory as electroweak theory, which is now commonly called Standard Model of particle physics after including the strong nuclear force. In electroweak theory Weinberg and Salam explicitly used the Higgs mechanism to generate the masses of force carriers of weak nuclear force, since it is a short range force while the electromagnetic force carrier was kept massless as it is a long range force. In the Standard Model not only gauge bosons acquire their masses through Higgs mechanism but all other massive particles like electron or quarks also get their masses by interacting with the Higgs field which is equally spread in the space. Salam-Weinberg theory (electroweak theory) is one of the most successful theories of particle physics. It has many important predictions besides the presence of Higgs field and its related Higgs particle. The theory predicted some new kind of force carrier (gauge boson) which has no change like photon but is mass like Wbosons, it is called Z boson. In early 1970s, the new particle Z boson and its related force mediation (current) was discovered at CERN. Consequently in 1979, Sheldon Glashow and Steven Weinberg of USA and Abdus Salam of Pakistan were awarded by the Nobel Prize in physics.

The last missing piece of the Standard Model was the Higgs boson and finally, 50 years after its prediction, it was observed on July 04, 2012 in Large Hadron Collider at CERN, which is a 27 Kilometer long underground machine (particle collider) at the border of Switzerland and France. The properties of the observed new particle are being analyzed by the scientists all around the world and it is found that this particle has exactly the same properties as were predicted by Salam-Weinberg theory or so called the Standard Model. The discovery of Higgs boson is a great triumph of theoretical physics and with its discovery, the Standard Model is complete and most successful theory till date. The Standard Model of particle physics has won around fifteen Nobel Prizes in Physics and last one is this years prize given to Englert and Higgs for establishing the BEH mechanism.
To conclude, we must say that Abdus Salam has played a vibrant role in current understanding of our universe. Salam was not only one of the greatest scientists in the last century, his role and services for the promotion of science and technology in the third world countries is also unmatched. He is founding father of Third World Academy of Sciences (TWAS) and the Abdus Salam International Centre for Physics (ICTP), Trieste, Italy, which is one of the leading institutes in the world, where students and scientists from the third world country visit to learn new and state of the art research in Physics. We end this essay by one of Abdus Salam’s quotations, “Scientific thought is the common heritage of mankind.”
References
[1] Y. Nambu, “Axial vector current conservation in weak interactions,” Phys. Rev. Lett. 4, 380 (1960); Y. Nambu and G. Jona-Lasinio, “Dynamical Model of Elementary Particles Based on an Analogy with Superconductivity. 1.,” Phys. Rev. 122, 345 (1961).
[2] J. Goldstone, “Field Theories with Superconductor Solutions,” Nuovo Cim. 19, 154 (1961).
[3] J. Goldstone, A. Salam and S. Weinberg, “Broken Symmetries,” Phys. Rev. 127, 965 (1962).
[4] F. Englert and R. Brout, “Broken Symmetry and the Mass of Gauge Vector Mesons,” Phys. Rev. Lett. 13, 321 (1964).
[5] P. W. Higgs, “Broken Symmetries and the Masses of Gauge Bosons,” Phys. Rev. Lett. 13, 508 (1964).
[6] G. S. Guralnik, C. R. Hagen and T. W. B. Kibble, “Global Conservation Laws and Massless Particles,” Phys. Rev. Lett. 13, 585 (1964).
[7] S. Weinberg, “A Model of Leptons,” Phys. Rev. Lett. 19, 1264 (1967).
[8] A. Salam, “Weak and Electromagnetic Interactions,” Conf. Proc. C 680519, 367 (1968).
Disclaimer: The Eqbal Ahmad Centre for Public Education (EACPE) encourages critical and independent thinking and believes in a free expression of one’s opinion. However, the views expressed in contributed articles are solely those of their respective authors and do not necessarily reflect the position or policy of the EACPE.
[contact-form][contact-field label=’Name’ type=’name’ required=’1’/][contact-field label=’Email’ type=’email’ required=’1’/][contact-field label=’Website’ type=’url’/][contact-field label=’Comment’ type=’textarea’ required=’1’/][/contact-form]