The James Webb Space Telescope (JWST) is the successor to the well-known Hubble Space Telescope (HST) that has amazed us with stunning images of the cosmos and greatly increased our understanding of it. It is a joint effort by NASA, ESA and the Canadian Space Agency (around 14 countries together). Named after the second administrator of NASA James E. Webb who played a major role in putting the first human on the moon, Webb is a step ahead of the Hubble, however, unlike the Hubble which mainly works in the visible light range of the electromagnetic spectrum, Webb will mainly take measurements/images in the Infrared region. The Webb will also be placed much farther than Hubble and it will have a much larger primary mirror than the Hubble. This fantastic project will revolutionise astronomy and space science in the coming decade.
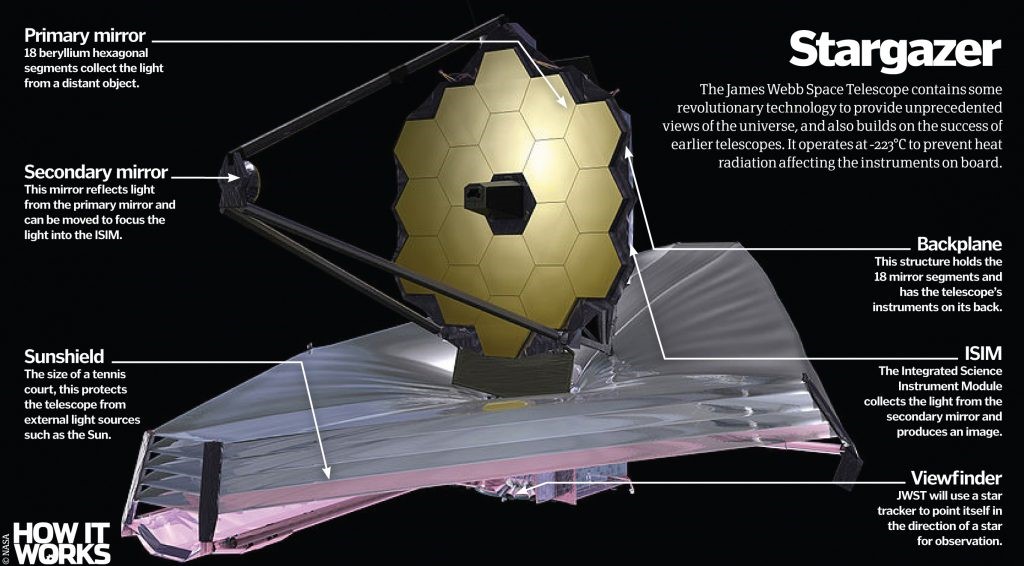
As seen in the image above, the telescope has a large primary mirror coated with gold, this mirror collects light coming from the farthest corners of our universe and directs it toward the secondary mirror mounted in front of it which then reflects it to the Integrated Science Instrument Module (ISIM) to create an image. A multi-layered Sunshield, the size of a tennis court is used to protect and cover the telescope from any heat or infrared radiations coming from the Sun, Earth as well as the Moon, it helps keep the telescope very cool (below 50 Kelvin or -220C). It has other equipment such as: A Viewfinder (star tracker), Solar Panels, Communications Antenna and a Spacecraft Bus (housing most of the steering and control equipment including an on-board computer and reaction wheels).
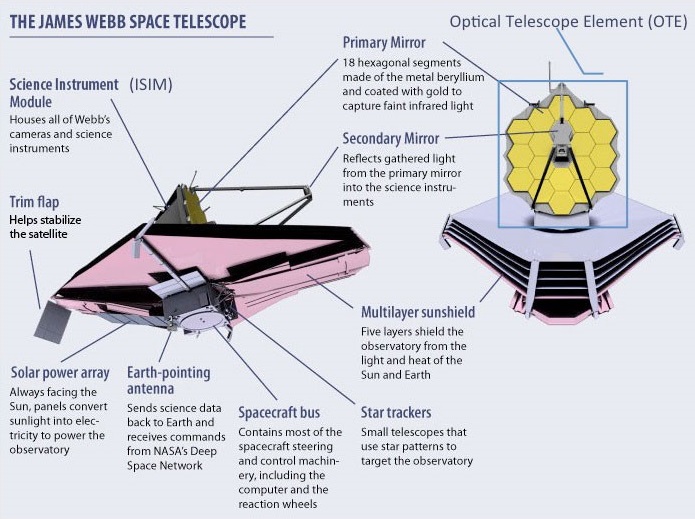
Main Science Goals
According to NASA,
Webb has four mission science goals:
-
Search for the first galaxies or luminous objects that formed after the Big Bang.
-
Determine how galaxies evolved from their formation until the present.
-
Observe the formation of stars from the first stages to the formation of planetary systems.
-
Measure the physical and chemical properties of planetary systems and investigate the potential for life in those systems.
Primary Mirror
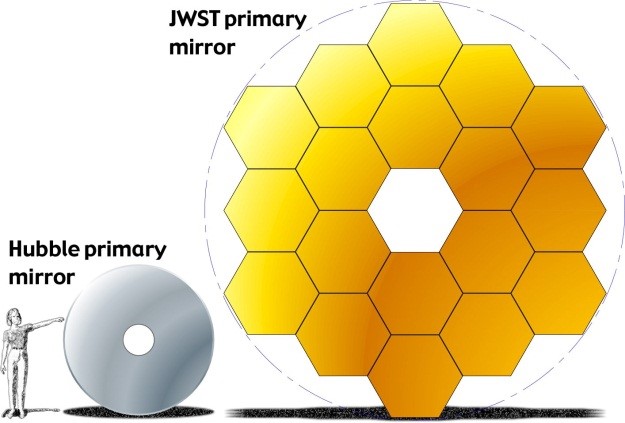
The Primary Mirror is composed of 18 hexagonal segments of smaller mirrors. The total diameter of this mirror is about 6.5m and is 2.7 times larger than the Hubble’s 2.4m mirror. A larger size mirror is needed to detect more light that would be coming from very distant objects, hence, higher sensitivity.
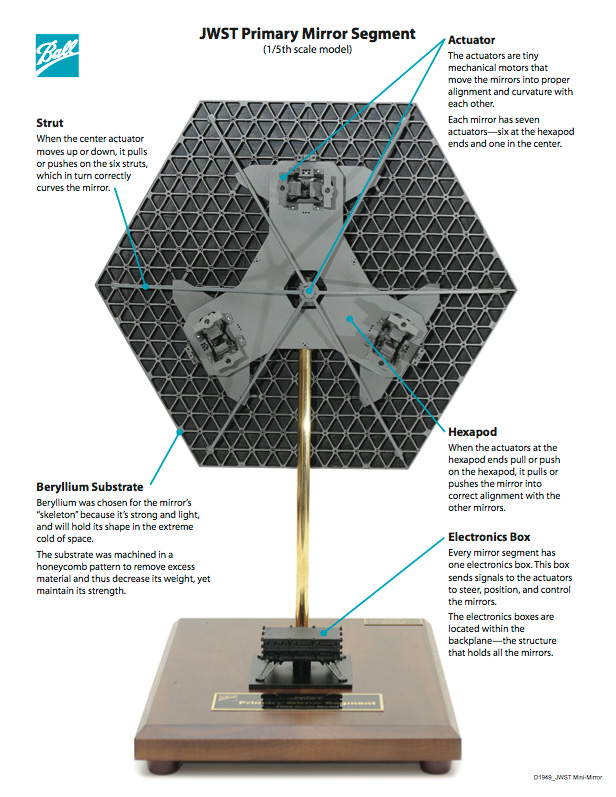
Segmented hexagonal sub-mirrors were used for high filling factor and a six fold symmetry. Since the telescope will be launched in an Ariane 5 rocket that has a smaller total diameter of 5.4m, the mirror had to be a folding one so that it would fit into the payload area which has a diameter of 4.57m. Each smaller segment of the primary mirror is made of Beryllium, a light-weight and strong metal that works perfectly in cold temperatures. It is coated with gold as it is a very good reflector of infrared light (as well as red/orange colours). The amount of gold used to coat the primary mirror is very small, about 48.25g, almost a golf ball worth of mass. The gold is then further coated with SiO2 or glass to protect it. Each individual mirror segment has actuators at their back that can fine-tune their alignment extremely precisely, to a degree of 1/10000 of a human hair. A technique called Wavefront Sensing is used to make all the segments act like a giant mirror. All 18 segments work as a single large mirror, focusing light precisely on the secondary mirror (which also has such mechanisms for alignment).
Backplane
This is the support structure for holding the primary mirror as well as the instruments module. A light weight strong structure made of graphite and other metal alloys. It will hold around 2400 kg of equipment while weighing around 500 kg itself. It is designed to be very steady for accurate detection by the mirrors, up to 1/10000th of a human hair or 32 nm.
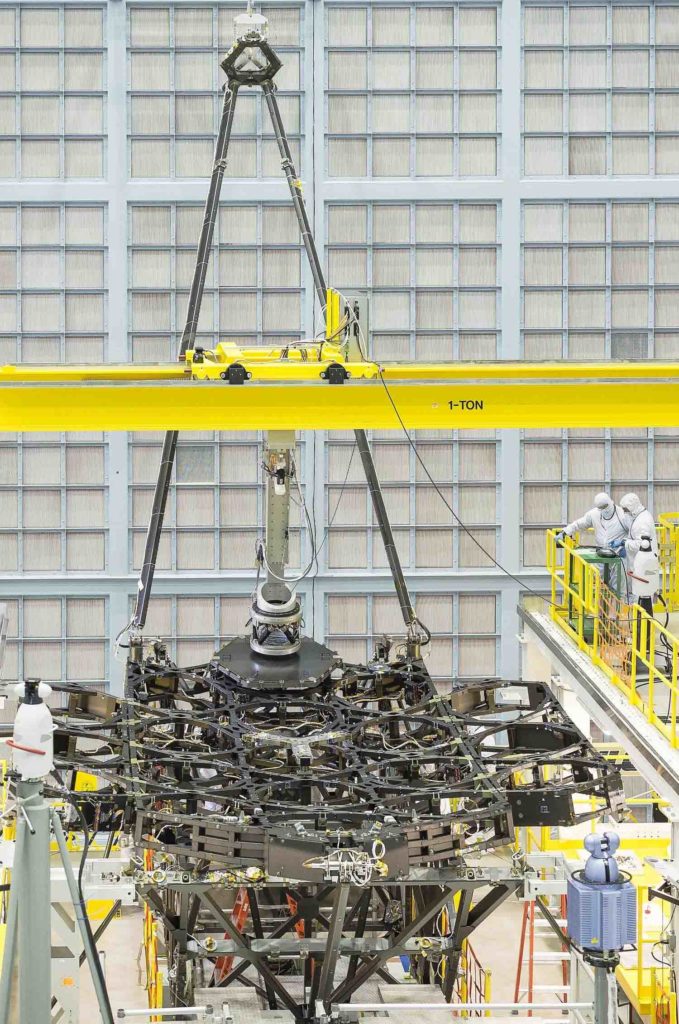
Integrated Science Instrument Module (ISIM)
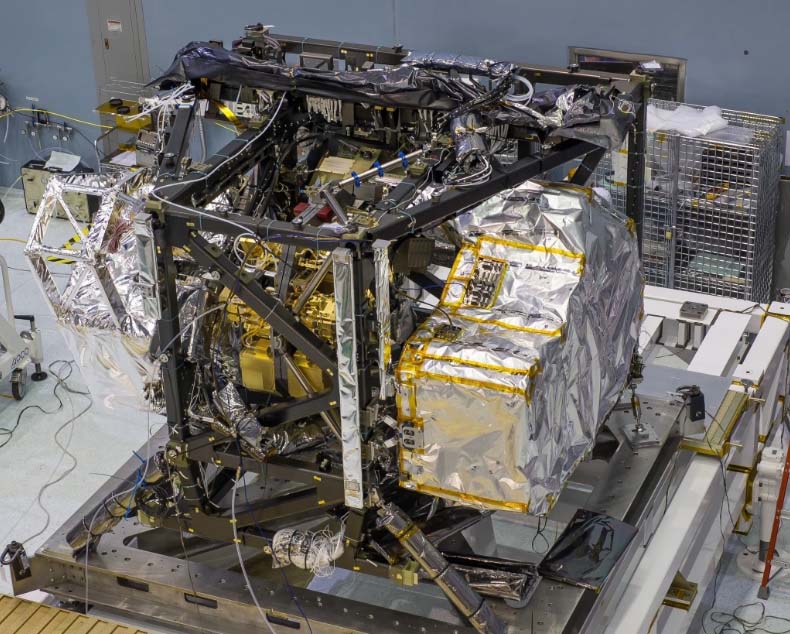
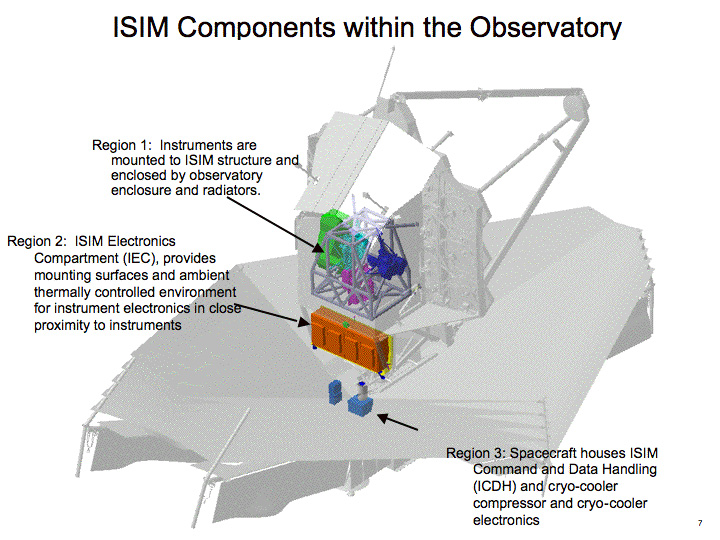
This is the unit at the backside of the primary mirror that houses all the sensors of JWST. It mainly has four instruments namely: Near-Infrared Camera (NIRCam), Near-Infrared Spectrograph (NIRSpec), Mid-Infrared Instrument (MIRI), Fine Guidance Sensor (FGS) combined with Near Infrared Imager and Slitless Spectrograph (NIRISS). As their names suggest, these are various types of detectors and spectrographs that work over a wide range of infrared wavelengths (0.6 to 27 micrometers). The guidance sensor is used to stabilise the line of sight of the telescope as well as its orientation and also adjust the fine steering mirror that can adapt to any jitter in the received light. Another innovative technology used is micro-shutters, these are small windows the size of a few hundred microns and they can be used to observe up to 100 different objects of interest simultaneously. Another interesting part used for MIRI is a Helium based cryocooler, that keeps MIRI cooler to 7 Kelvin (-266C) since it has to detect longer infrared radiations in the range of 5 to 28 microns.
Sunshield
When we talk about infrared, we must remember that these waves are actually heat radiations. The Webb uses a multi-layered sunshield that blocks/reflects the Sun’s solar radiations as well as any radiations coming from the Earth and the Moon. This is extremely important as the highly sensitive infrared detectors need to be in a very cool environment to take accurate measurements, any infrared interference from the Sun, Earth, Moon or even the on-board equipment can interfere with the readings. It is the same as looking at something while it is placed between the Sun and your eyes; it is almost impossible to see the object until you block the Sun’s light with your hand and then see the object easily. The telescope will be placed on the other side of the sunshield and it will be in very cool temperatures. In the diagram below, it can be seen that the sunshield will always face the sun as the Webb revolves around the Sun-Earth system at a fixed point called the Lagrange point L2.
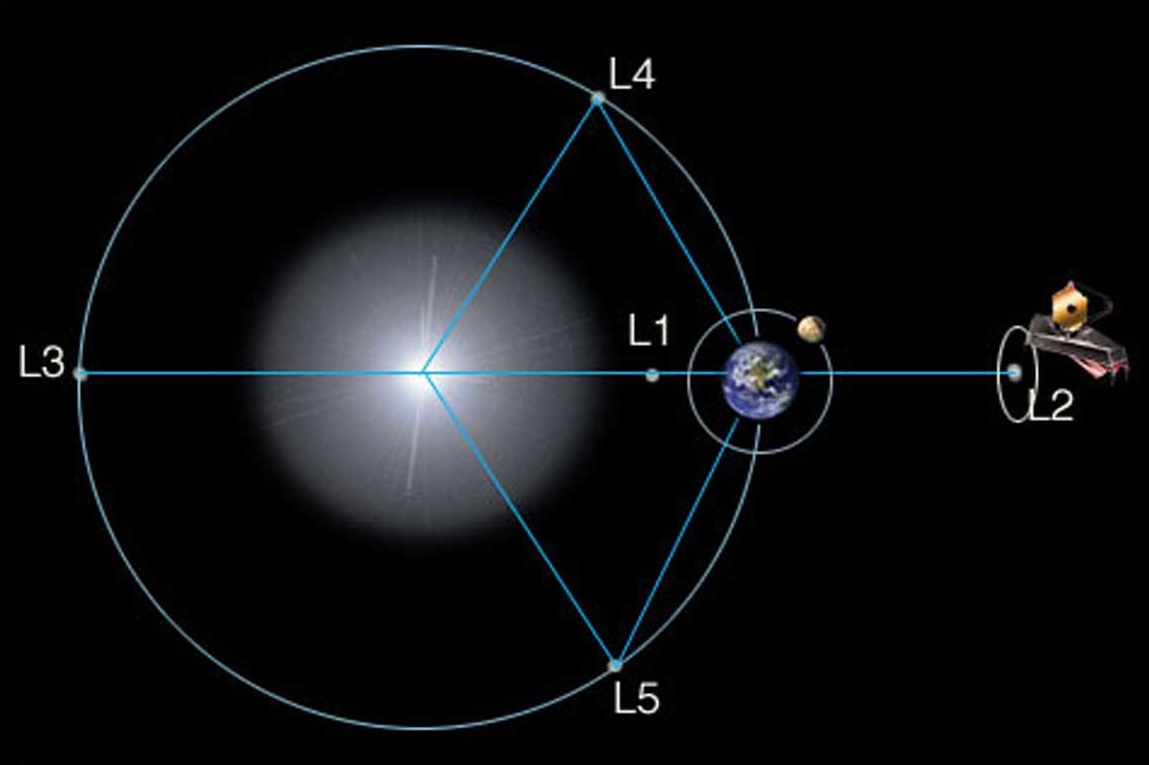
As seen in the image below, the sunshield is an extremely thin sheet made of a material called Kapton. It is also coated with aluminum and in first two sun facing layers, Silicon, for extra durability. Kapton is a polyamide and has very high thermal resistance; it is very stable at a wide range of temperatures from -269C to 400C. The five layers are separated from each other so that heat conduction between each layer may not take place, vacuum between them acts as a very good thermal insulator.
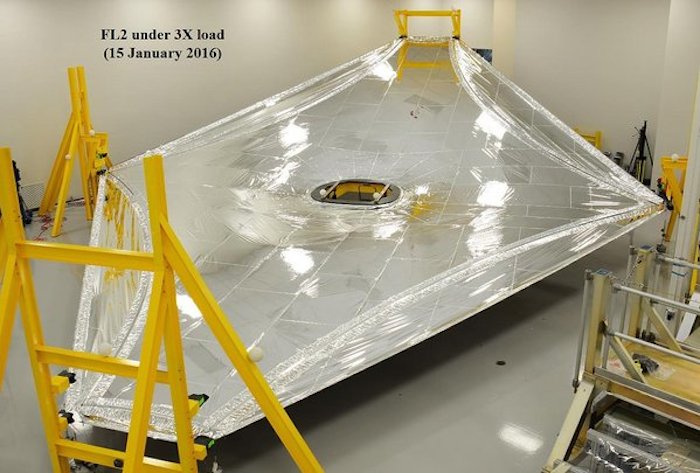
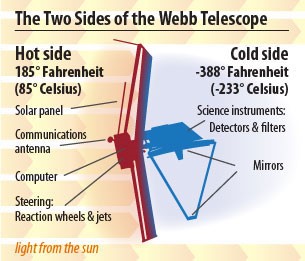
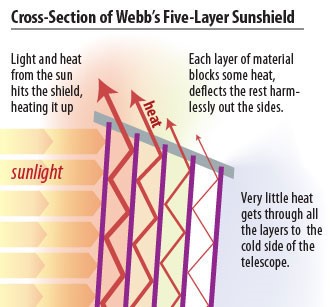
Why Infrared?
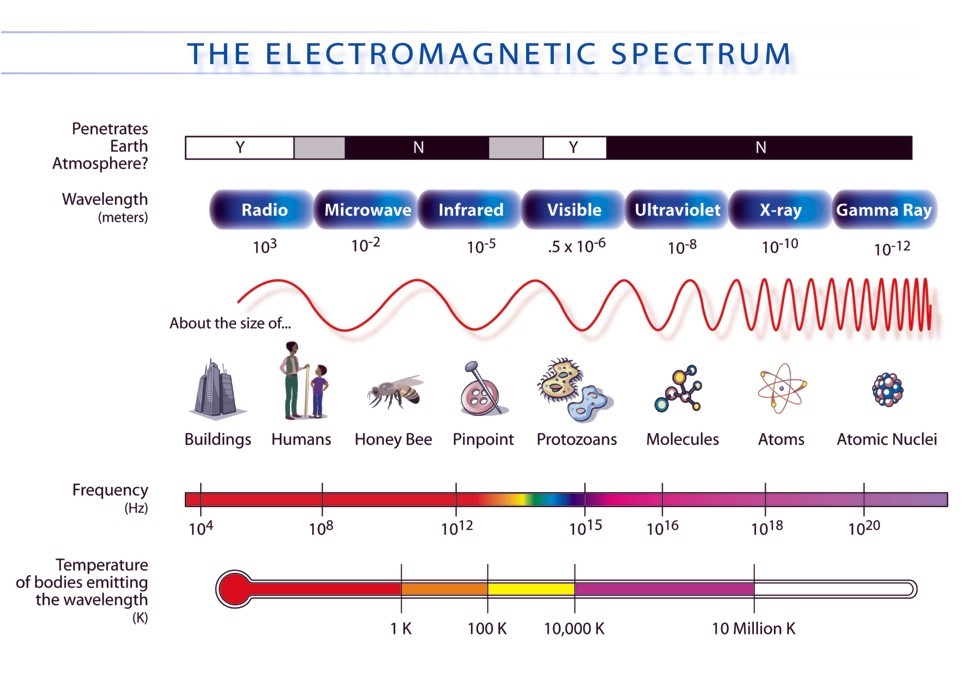
Unlike the Hubble that mainly dealt in visible light, Webb uses infrared light mainly. There are two major reasons for this.
First, as seen in the image below, huge dust clouds in the cosmos can obscure many stars/planets, if we take the same image with infrared, many hidden stars/planets become visible.
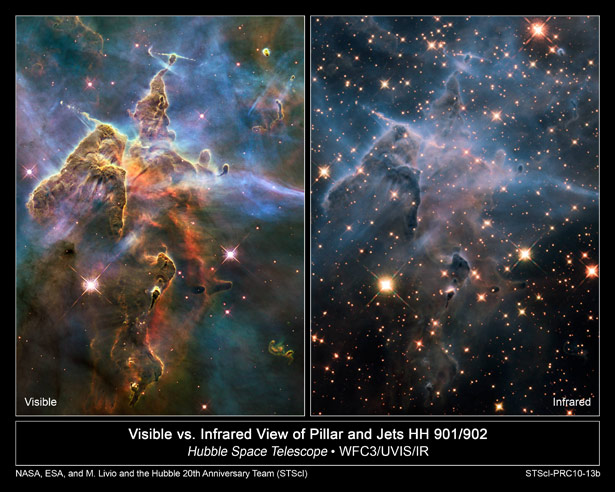
Second, since Webb will be used to study galaxy formation as well as star and early planetary formation soon after the big bang, the light that has travelled towards us will be highly redshifted well into the infrared region. This is due to Einstein’s General Relativity Theory which says that as the universe is expanding, space is also stretching; light’s wavelength will get longer and longer as it travels through enormous distances. This phenomenon is called redshift and it is akin to the Doppler Effect observed when a siren approaches and recedes from an observer. As the siren gets nearer, the sound gets louder and frequency gets higher indicating a decrease in wavelength, as it passes the observer and gets farther, the noise subsides and its frequency gets smaller indicating that the wavelength has got longer.
Light that emanated from the early times of our universe has travelled through billions of years and millions of light year distances, hence, its wavelengths will have considerable redshift i.e. enlargement or shift towards the infrared region of the electromagnetic spectrum.
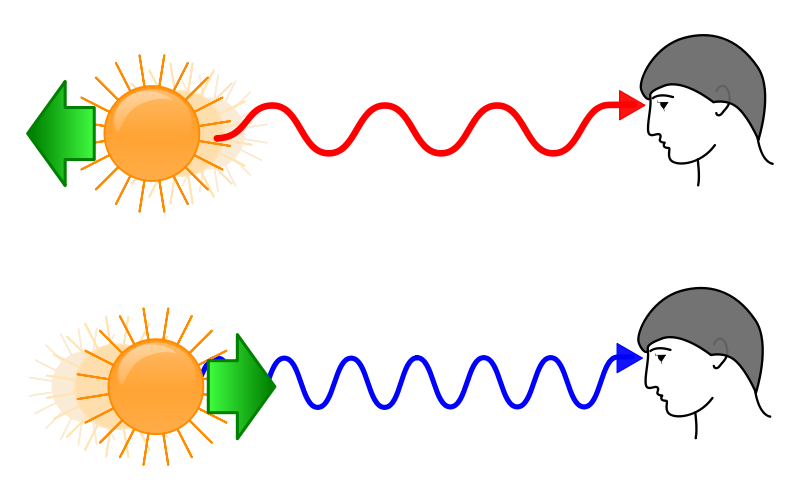
Spectral analysis is done for various objects in the universe, by comparing the observed spectrums with those of known spectrums, we can determine the amount of redshift present. A redshift of 1 would mean that the spectral features would have shifted to twice that of its wavelength. If the features shift to three times longer wavelength then it is redshift 2 and so on. Webb can measure redshifts from 15-30. The image below shows redshift in an observed spectrum compared with a rest or normal spectrum. All the peaks for various molecules/compounds have shifted towards the right, greater wavelengths.
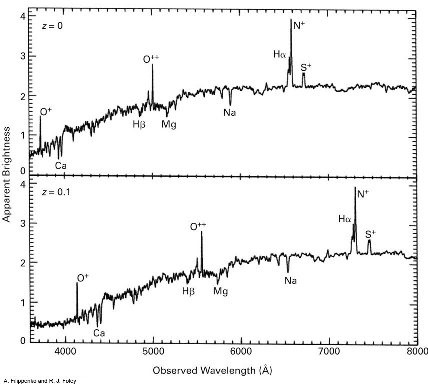
Early light could have started in the visible or high energy ultraviolet side of the spectrum. Therefore, if we want to peek into the past and observe how the first galaxies, stars and planets formed, we have to observe in infrared. It is amazing that the light we see today might have taken millions if not billions of years to travel and things back at its originating point would be totally different now.
Why Lagrange Point 2 (L2)?
The Lagrange Point 2 or L2 is a location in space around 1.5 million kilometers away from Earth. To make a comparison, the moon is about 385000 km away from Earth while the Earth is around 150 million km away from the Sun. The Hubble Space Telescope is in low Earth orbit at about 570km. It will take Webb around 30 days to reach L2.
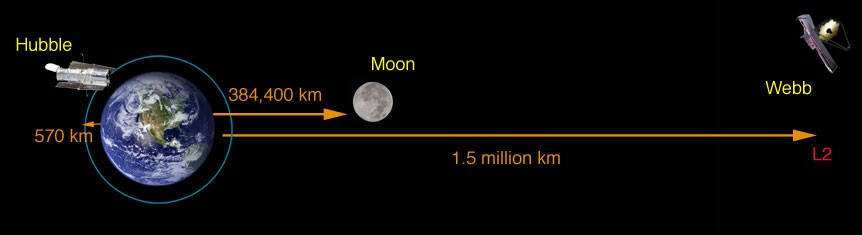
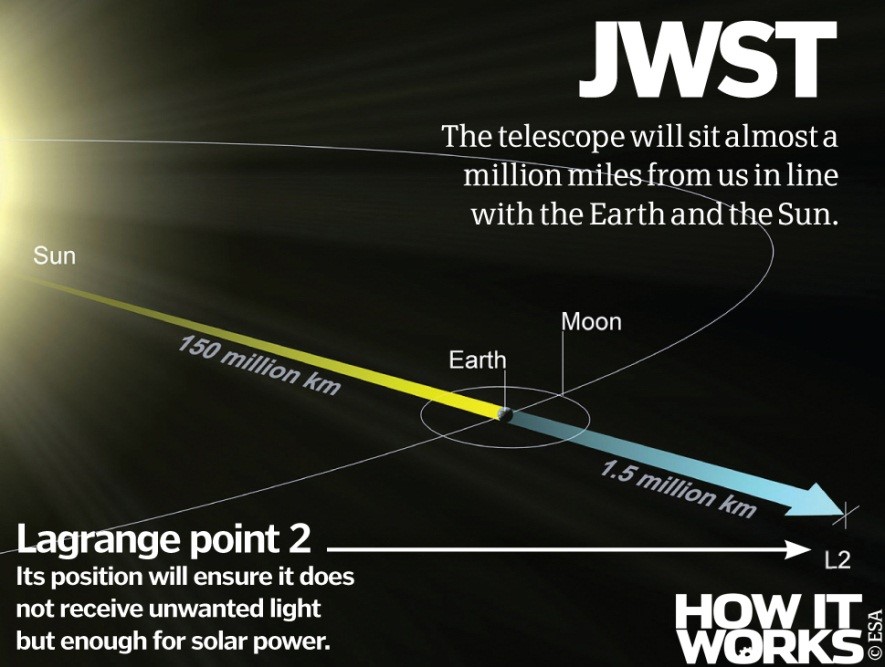
So why was L2 chosen for Webb? We needed a place in space that would be far away from the Earth and Moon so that any infrared noise/interference by them may not affect the highly sensitive readings taken by Webb’s instruments. The Earth’s atmosphere absorbs most of the infrared radiations coming from space and also emits infrared radiations (basically heat) from its surface, thus, blinding highly sensitive infrared sensors. At high infrared wavelengths, even the heat emitted by a sensor can interfere with the readings. Space is an ideal location to detect even the faintest of infrared signals.
We also wanted Webb to stay in constant line of sight with the Earth for communication purposes and the Sun for its solar powered systems. However, the farther an object is away from the Sun, the longer it takes to orbit according to Kepler’s third law of harmonies and that would create a difference in orbital time/locations of Earth and Webb. The good thing about L2 is that in the Sun-Earth system (we can ignore the Moon’s effect for now), when the Sun and Earth is aligned with each other, their combined gravitational pull is larger and it will make Webb orbit at a faster speed, constantly aligned with the Sun-Earth. Thus, Webb will actually orbit the Sun instead of orbiting the Earth as shown by the figure below.
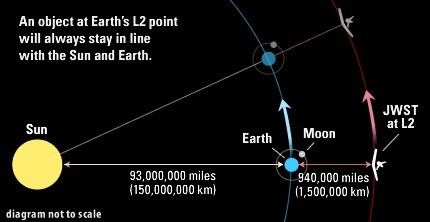
It must also be noted that Webb will be in a halo orbit around L2 as shown nicely in this YouTube video: https://www.youtube.com/watch?v=IyyQqaF4tNY. The halo orbit is such that Webb will orbit L2 almost perpendicular to the Sun-Earth elliptic. This is to avoid the shadow of the Earth or Moon and allow the solar panels on Webb to generate electricity non-stop. It will also allow the Webb to be in constant communication with the Earth through NASA’s Deep Space Network (Antennas located in Australia, Spain and California), this was not the case with Hubble as it used to go in and out of the Earth’s shadow every 90 mins.
Launch
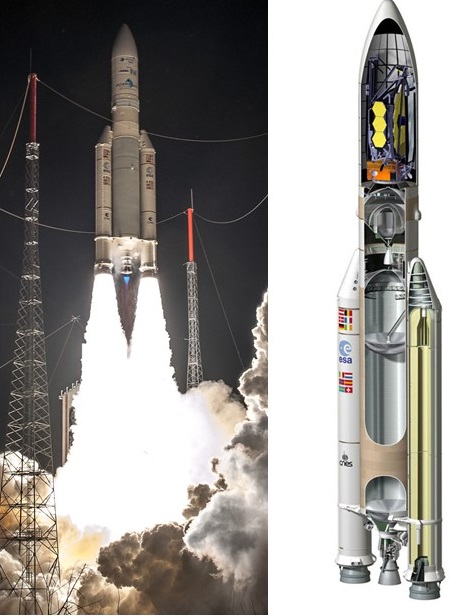
James Webb Space Telescope is destined for launch in spring 2019. It will be launched in an Ariane 5 rocket from the European Spaceport near Kourou, French Guiana (located near the Equator in South America). The European Space Agency is providing the launch vehicle and other facilities. This location was chosen as it lies near the Earth’s equator, making it easy to launch a rocket with the maximum push/velocity from the Earth, placing it relatively easily in a geostationary orbit. This launch site has the open sea to the East making any debris or objects falling down into the sea instead of human settlements.
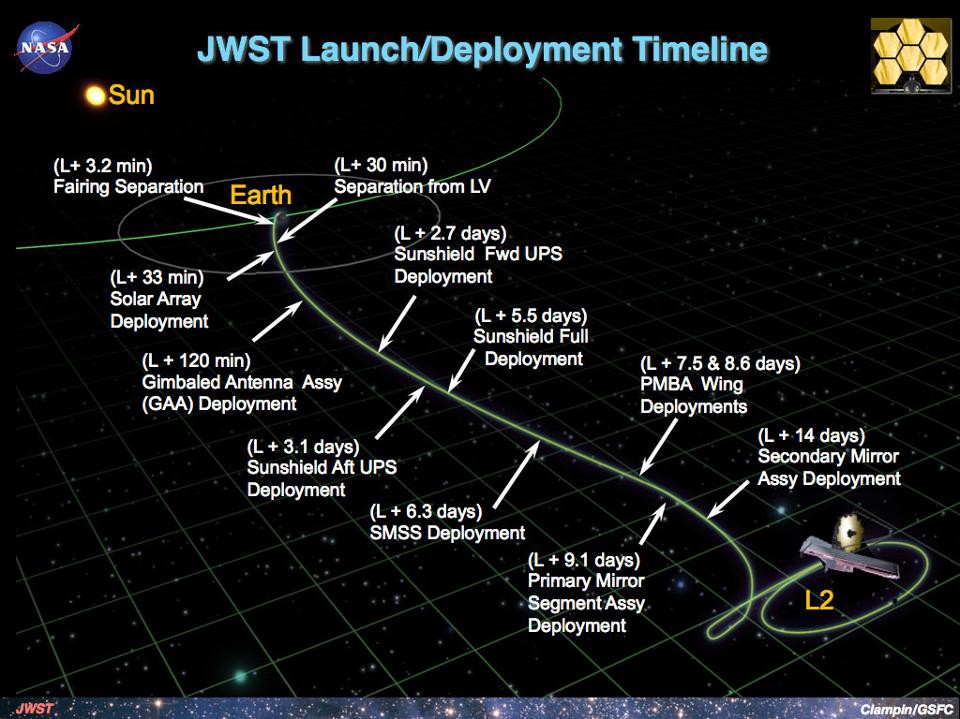
How much in the past can Webb see?
The diagrams below illustrate how far back in time Webb can see. Webb can see approximately 200 million years after the Big Bang. That is quite back in time, considering that the universe is around 13.7 billion years old, hence, we can effectively see 13.5 billion years into the past and observe how early galaxies and stars/planets were formed.
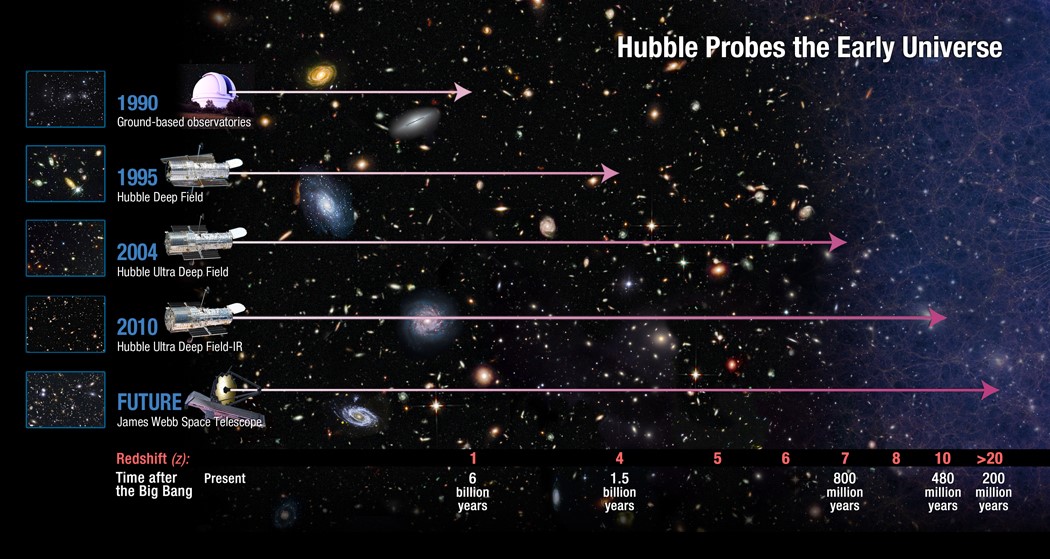
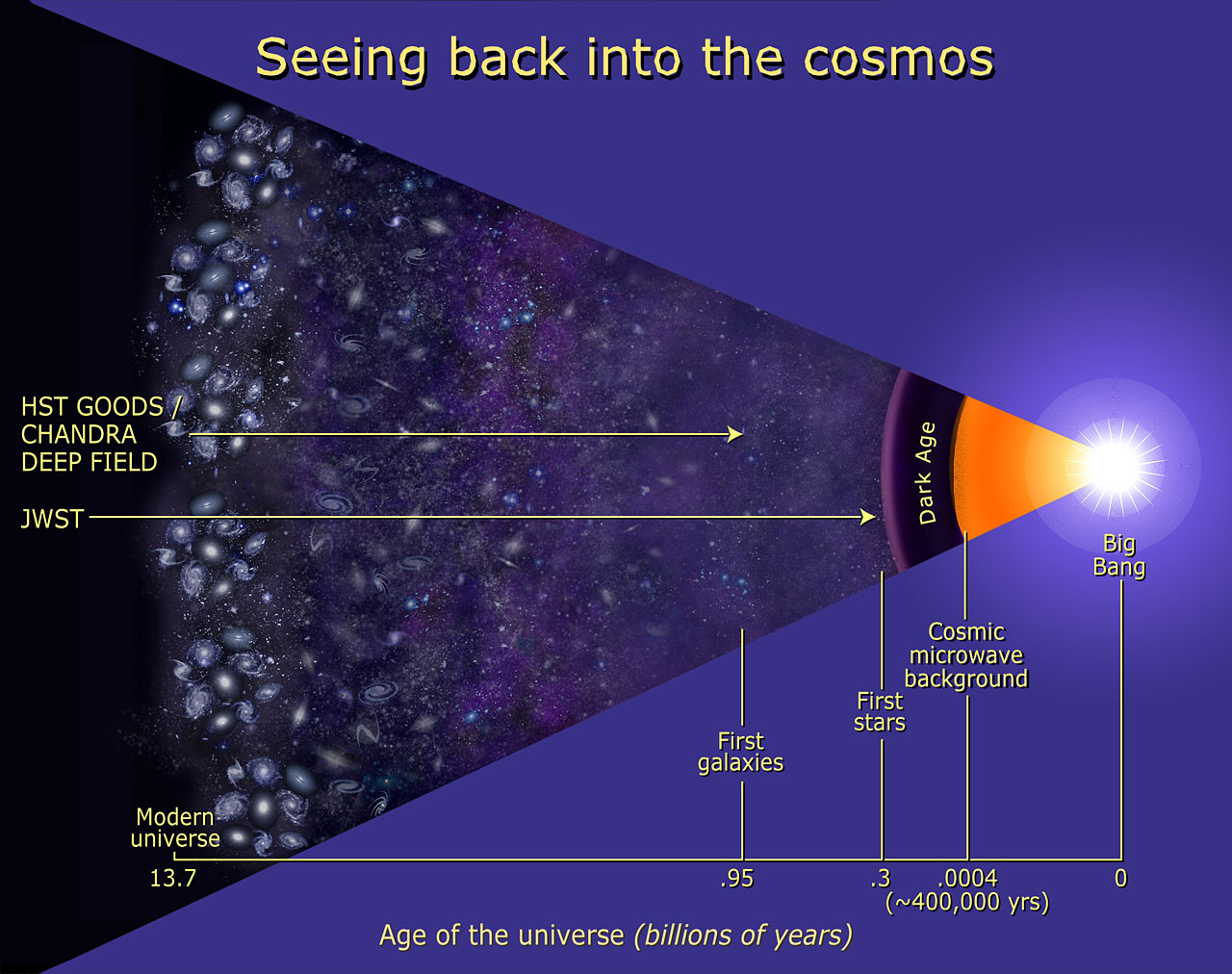
Lifespan of the JWST
The Webb is designed to perform its work for 5 years guaranteed after becoming fully operational at the end of a 6 month long commissioning process. It will have fuel for 10 years though. The lifetime of Webb is limited due to the amount of fuel it has to store for occasional orbit corrections and maneuvers as the L2 point is semi-stable, which means that an object at that location will eventually drift away from it due to the gravitational attraction of other objects in the solar system and beyond.
References/Credits:
National Aeronautics and Space Administration (NASA)
European Space Agency (ESA)
Space Telescope Science Institute (STScI)
James Webb Space Telescope (Wikipedia)
Northrop Grumman
HOW IT WORKS
Arizona State University (ASU)
Institute for Astronomy (IFA), University of Hawaii (UH)
Amazing Space
YouTube
About the Author:
Shahbaz Aslam Khan is a Development Engineer and teaches at Physics Department of Lahore University of Management Sciences (LUMS).
He can be reached at shahbaz.aslam@lums.edu.pk
Someone has made effort to write an informative piece, however, it appears to be more of a copy paste and thoughtless effort. Author has missed the required analysis on usefulness of the telescope in future and comparative study with existing space telescopes with their capability. An experienced professional might have given a much more in depth detail on the subject. Being naive, I am fully aware to be not expecting such from a Development Engineer at this stage, but this website is more capable to achieve than this.
Jawad Ashraf why are you criticising and sounding so jealous? I think it is a good effort and summarised the project nicely in an easy to understand manner. Of course the author is young and had to get the knowledge from somewhere. You must encourage such things and also do your part.
[…] This article was published on the website of Eqbal Ahmad Centre for Public Education (EACPE): James Webb Space Telescope […]